By Beth Davenport
Antibiotic resistance is one of the world’s biggest health crises. In fact, like climate change, it’s one of the only crises that is predicted to worsen in the future, instead of improving with scientific research and innovation. The good news is, Beth Davenport is going to explore here the possibility of killing two birds with one stone; there is a solution that can mitigate both environmental and health crises.
What is antibiotic resistance?
To provide some microbe background, bacteria are single-celled organisms that are found everywhere (literally everywhere); there are more bacteria than human cells in our bodies. Lots are good bacteria, playing important roles in our metabolism and immune system, or environmentally cycling the world’s elements to ensure our survival. But a tiny fraction of bacteria are bad for human health. We all know this of course – try and name someone who hasn’t been prescribed antibiotics to fend off strep throat, or perhaps an unfortunate STI at some point in their life!
At a basic level antibiotic resistance is the development of resistance in bacteria towards antibiotic drugs, that were once able to kill them, or prevent their growth. Usually, this is through acquiring a resistance gene. If you are interested in knowing a bit more about the scientific mechanisms of resistance, keep reading. If not, feel free to skip to the next section!
Antibiotics are usually orally taken, systemic drugs (meaning they are absorbed through our gut and can circulate in our blood system to access our entire body). Unlike Trump’s suggestion, we cannot just drink disinfectant solutions to rid ourselves of bacterial infection, as it is toxic to our own cells too. Antibiotics, on the other hand, target things in bacterial cells that are required for their survival, that don’t exist in our own human cells. This is the key to why antibiotics are so effective. For example, inhibiting bacterial cell wall production (our cells don’t have cell walls), is a popular target for many well-known antibiotics such as penicillin, cephalosporin and vancomycin. While penicillin and cephalosporin block the builder (enzyme) that builds the cell wall, vancomycin blocks the little bricks (dipeptides) that are needed for the builder to make a cell wall in the bacteria. A bacterium without a cell wall bursts and dies. Success.
So, what are the mechanisms for bacteria becoming resistant to antibiotics? Usually, it involves the bacteria acquiring a resistance gene. Here are a few fun examples, focusing on the cell wall building inhibitors I’ve already talked about. A resistance gene for penicillin encodes a protein that cuts the penicillin drug like a pair of scissors, so it is no longer the same shape and can’t bind the builder of the cell wall. A resistance gene for cephalosporin encodes the same builder enzyme for the cell wall, but with a slightly changed shape so that cephalosporin can’t recognise the builder to stop it. Finally, a resistance gene for vancomycin encodes a new type of brick for the wall, which the builder can still use, but that vancomycin can no longer recognise. Some strains of bacteria can contain all of these resistance genes at once, termed ‘superbugs’. Examples of these superbugs are becoming more prominent in the news – I bet you’ve heard of the multi-drug resistant MRSA, or the super strain of gonorrhoea which is virtually resistant to all treatments.
How does antibiotic resistance spread?
The use of antibiotics selects for resistant bacteria strains in two ways. Firstly, if one bacterium has a DNA mutation in a bacterial population that makes it slightly resistant to a drug, then exposing the population to a little bit of the drug will kill all but that one mutant bacteria. Et voila – this bacterium multiplies and suddenly we have an entire resistant population. Secondly, bacteria can exchange DNA between themselves with the use of tiny syringe-like contraptions. A (slightly uncomfortable) human analogy would be me (brown-eyed) touching my finger to your arm, my fingertip skin unpeeling to reveal a syringe, which gouges a hole into your arm, and injects one of my genes into you. Suddenly, your eyes change colour from blue to brown. Weird huh? Anyway, the point is, a bacterium that has the resistance gene for an antibiotic can just pass it by microscopic syringe to any other bacteria it happens to come across. (Just to be clear, this choice of human analogy would not be welcome in scientific literature, something like that could definitely not happen if you implanted a syringe in your finger, but it makes it more engaging right?)
Basically, antibiotic overuse promotes the bacteria-syringe activity to spread a resistance gene to as many different bacteria as possible. Hence why doctors and other health care professionals are attempting to reduce the amounts of antibiotics prescribed for inappropriate cases – it should be a last resort treatment if an immune system can’t handle an infection on its own. It’s also why you may get a friend nagging you to ‘always finish your course of antibiotics’ when you’re back from the doctor with a diagnosed UTI and a packet of pills. This makes sure the whole bacterial population is killed by exposing it to enough of the drug, making sure there is no slightly resistant one alive to replicate.
Antibiotic resistance as a global crisis
Antibiotic resistance has been reported for almost all antibiotics, usually a few years after the drug is used on a wide scale medically. This would not be such a problem if we kept discovering new antibiotics to replace the ones resistance has arisen against. But almost all the classes of antibiotics we currently use were discovered in the 1950s – the drug companies found the ‘low hanging fruit’ so to speak, and inventing new drugs in the lab, or discovering new antibiotics in nature is becoming rarer and rarer. (As a side note, most antibiotics are based on natural products, and the progressive destruction of habitats rich with biodiversity is not exactly helping the effort to source new drugs). Meanwhile, resistance genes are spreading at ever-increasing rates. So rather than the existence of resistant bacteria being the problem, it is really the combination of increasing the spread of resistance worldwide with antibiotic overuse, and the lack of progress in antibiotic drug discovery that is causing the global crisis.
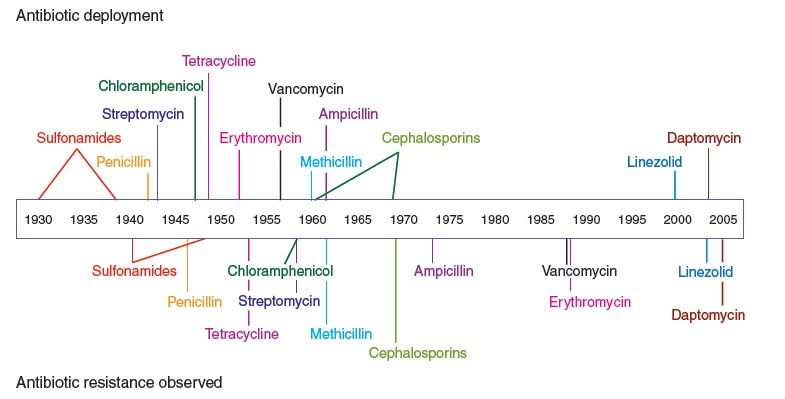
Timeline of popular antibiotics’ deployment in medicine, and the subsequent evolution of resistance to each one. Source: paper from Nature.
Antibiotics are cheap, safe and effective ways to treat bacterial infections that were once deadly. For example, they enable surgeries where the risk of infections are high such as organ transplants, they allow the use of certain cancer treatments, they make caesarean sections possible, they have drastically increased survival rates of diseases such as Tuberculosis and Malaria, the list goes on. Today, antibiotics are taken for granted, but they are responsible for enabling a lot of modern medicine that we have enjoyed over the last eight decades, especially in the UK. Just like with fossil fuels, post-WW2 civilisation has a dangerous addiction to antibiotic drugs that will ultimately end in disaster.
Antibiotic resistance has scary consequences: drugs that were once lifesavers will become utterly useless. It is already estimated to cause 700,000 deaths a year, but a review commissioned by the UK government and Wellcome Trust has predicted that this will rise to 10 million by 2050 (and if you are wondering the cost of all this globally, it’s estimated at a modest $100 trillion).
What are the ways of overcoming antibiotic resistance?
There are various solutions that have been proposed to this crisis. A lot of them are mundanely obvious of course, and as I am currently writing this in a global pandemic, I won’t risk them triggering you (but remember to wash your hands). Others are biotechnological solutions to replace antibiotics – if you’re interested, you should check out phage therapy (the Soviets realised the potential of this technology before us – read more here).
The reason I am writing about antibiotic resistance, despite usually focusing on climate change, is because there is a frustratingly easy way to reduce antibiotic resistance spread that also helps to prevent the worst climate change scenarios from becoming true.
I’m guessing you already know the vast array of reasons in support of plant-based diets to mitigate climate change, so I won’t go into it (but here’s a link in case). But did you know that around two-thirds of the entire global use of antibiotics is given to livestock? (In fact, the US uses 80% of its antibiotics in livestock). Why? Because it’s a cheap way of promoting animal growth, and preventing bacterial infections spreading in crowded meat and dairy farms. What does this mean for human health? It means we are providing an ideal environment for bacteria to spread resistance genes about and make new superbugs.
Resistance can then get transmitted directly or indirectly to humans (see the diagram below). In fact, up to 90% of livestock antibiotics are excreted in urine or stool, which can then be widely dispersed through fertilizer, groundwater, and surface runoff. This means that even in the soil, bacteria are busy syringing resistance genes to their neighbours to ward off drug attacks in the environment. A scary example of livestock antibiotic resistance at work can be seen in the spread of a new superbug: livestock-associated MRSA, or LA-MRSA (you can read more here).
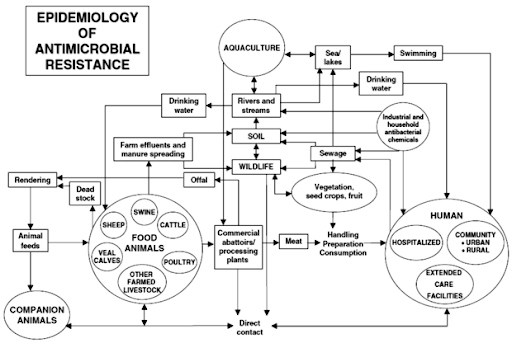
In 2015, a paper was published that was the first to attempt quantifying global antimicrobial consumption by livestock. Antibiotic amount used in livestock in 2010 was globally estimated at 63,151 tonnes. By 2030, this is projected to reach 105,596 tonnes worldwide – an increase of 67%.
Consider these facts: meat consumption has grown almost twice as fast as the human population in the last 60 years; the total biomass of animals raised for food now outweighs that of humans and wildlife combined. This means new drug-resistance mutations are more likely to spread, or new combinations of resistance genes will be syringed together, in the livestock reservoir rather than in humans.
Interestingly, the same research team a few years later modelled what this increase would look like if we implemented meat consumption reduction:
“ Limiting meat intake worldwide to 40 g/day—the equivalent of one standard fast-food burger per person—could reduce global consumption of antimicrobials in food animals by 66%.”
In comparison, the FAO estimates the average daily meat consumption in the United States at around 120g, and the UK about 85g.
But why reduce meat consumption? Can’t we just stop giving them antibiotics for growth promotion? The short answer is yes, we can reduce antibiotic use in livestock with top-down policy interventions. Regulations have recently been implemented in the EU especially, (though Brexit may allow the UK to skirt the new wave of regulations – read more here). But we will always need some antibiotic use for preventative medicine if livestock continues to exist at their current scale for our consumption. They are, at the end of the day, so like us in biology that they are infected by the same bacteria, and suffer the same consequences without the drugs when living in crowded conditions.
The question then becomes: is it morally justifiable to allocate a fraction of our finite provision of effective antibiotics to animals that are raised for food? Ironically, reducing meat consumption isn’t mentioned on global health organisations’ homepages about the antibiotic resistance crisis, despite consistently listing all other potential solutions. This phenomenon is mirrored in climate change literature – the meat and dairy industry’s contributions to greenhouse gas emissions are often left off from information campaigns (I urge you to watch the Cowspiracy documentary - I will never feel the same way about Greenpeace).
Ultimately, reducing meat consumption is the logical route to take to address this crisis; on top of causing the highest possible reduction in antibiotic resistance spread, it has huge health benefits and reduces a hefty amount of greenhouse gas emissions to help mitigate the oncoming climate crisis. Oh also, it might be worth mentioning that with decreased meat consumption we reduce risks of future pandemics. I certainly don’t want to go through all this again any time soon.
Some conclusions
My hope is that this argument for decreased meat consumption is more black-and-white for those struggling with the distant nature of the climate crisis. Eating meat doesn’t just indirectly kill or induce suffering of other humans and animals through contributing heavily to climate change, but it also causes death more directly in a shorter timeframe – and the amount of death is set to increase dramatically.
I want to make it clear that antibiotic resistance is a HUGE topic to write about – there are so many approaches I could have taken. As I am a molecular biologist, a science-communication style article felt most befitting, as I feel I have the appropriate knowledge to do so. However, the social science of antibiotics is complex and fraught with inequalities, especially on a global scale, like so many health crises we have experienced. I am aware that generalising global meat consumption is not fair, just as blaming those who emit next to no emissions for climate change is ridiculous. Unfortunately, bacteria do not stick to political borders, so whatever resistance is generated in one place, will surely spread to all the others.
It does remain clear, however, that those in Western countries who have so far enjoyed the highest daily meat consumption per capita, have a responsibility to turn to a plant-based diet. Hopefully, a wider awareness of the variety of negative consequences of eating meat will encourage further questioning of why anyone continues to do so.
Suggested reading
Scientific papers on livestock antibiotic use:
Van Boeckel, T. P., Brower, C., Gilbert, M., Grenfell, B. T., Levin, S. A., Robinson, T. P., ... & Laxminarayan, R. (2015). Global trends in antimicrobial use in food animals. Proceedings of the National Academy of Sciences, 112(18), 5649-5654.
Van Boeckel, T. P., Glennon, E. E., Chen, D., Gilbert, M., Robinson, T. P., Grenfell, B. T., Levin, S. A., Bonhoeffer, S., and Laxminarayan, R. (2017) Reducing antimicrobial use in food animals. Science, 357, 1350–1352.
Tiseo, K., Huber, L., Gilbert, M., Robinson, T. P., & Van Boeckel, T. P. (2020). Global trends in antimicrobial use in food animals from 2017 to 2030. Antibiotics, 9(12), 918
Martin, Michael J et al. “Antibiotics Overuse in Animal Agriculture: A Call to Action for Health Care Providers.” American journal of public health vol. 105,12 (2015): 2409-10. doi:10.2105/AJPH.2015.302870
Kirchhelle, C. (2018). Pharming animals: a global history of antibiotics in food production (1935–2017). Palgrave Communications, 4(1), 1-13.
A great blog article about the Van Boeckel scientific paper in 2017:
https://cddep.org/blog/posts/global-plan-cut-antimicrobial-use-animals/
CDDEP global antibiotic resistance partnership review on ‘the state of the world’s antibiotics’ 2015:
CDC (2019) Antibiotic resistance Threats Report
The independent Review on Antimicrobial Resistance, backed by the UK government and the Wellcome Trust and headed by the economist Jim O’Neill:
Antibiotic resistance and potential of vaccines in restricting the need for antibiotics:
Phage therapy paper:
Easy read for climate change and antibiotic resistance as both environmental reasons to stop eating meat:
LA-MRSA investigation in UK farms:
The WHO main page about antibiotic resistance that fails to mention reducing meat consumption:
Regulations across the globe for antibiotic use in livestock:
Comments